The basic capacitance multiplier circuit is essentially a simple emitter follower with a capacitor on the base and a feed resistor from the input to the base to turn the transistor on. A capacitor from the base to ground provides the smoothing.
The capacitance multiplier circuit operation is quite straightforward. It acts as a simple emitter follower. The resistor R1 provides bias for the base emitter junction, and the capacitor provides smoothing. This considerably reduces the levels on noise on the output, i.e. Vout.
The effect of placing the transistor in the circuit is that it effectively multiplies the capacitance on the base by the current gain of the transistor, i.e. by β
The capacitance multiplier circuit is not a voltage regulator. The output voltage varies directly with the input Vin as there is no voltage reference. Generally the output voltage is about 0.65V less than the base voltage, and around 2 – 3 V less than Vin when a load is applied.
The ripple and noise levels on the output can be reduced to very low levels> Increasing the values of R1 and C1 reduce the output ripple, and increasingly at low frequencies. On the downside large values of R1 and C1 cause the output to rise slowly towards the required value after turn on, because of the large time constant of R1 and C1.
Modified capacitance multiplier
The drawback of the circuit is that in its basic form, there is very little voltage drop across the series pass transistor, and noise reduction is not as high as it may be. To overcome this, some people place a resistor across the capacitor and this provides a potential divider reducing the voltage at the base and increasing the voltage drop across the transistor. This enables it to provide better noise reduction, although it does increase power dissipation and reduces the voltage at Vout.
This version of the capacitance multiplier circuit includes an additional resistor from the base to ground to reduce the base voltage and provide additional voltage drop across the transistor for improved smoothing. This is more important when the levels of ripple are higher.
Typically the voltage through the potential divider should be sufficient to maintain the base voltage sufficiently. A judgement can be made regarding the level of current though the potential divider, but often in these types of circuits it may be ten times the base current. This would ensure that the emitter voltage is maintained over a wide range of output current levels.
Example application for a capacitance multiplier
The power supply shown here provides only smoothing at this stage and no stabilisation or voltage regulation. The input is taken from the mains and rectified by the bridge rectifier. It then passes into a smoothing capacitor, C1, to provide the first smoothing and remove the major ripple. This capacitor should have a large ripple current capability if the supply is to be used for high current levels.
It should be remembered that the capacitance multiplication effect can only be realised if there is a sufficient voltage drop across the series transistor. Typically this should be a minimum of 3 volts at all times.
The capacitor C2 is connected to the base of the transistor TR1. This provides the capacitance for the capacitance multiplication effect.
TR1 is the main pass transistor and must be able to drop the required voltage and at the required current, so power dissipation may need to be calculated.
On the output there is a capacitor to provide a little further decoupling and to ensure that the circuit remains stable. The resistor ensures the output voltage drains away at power removal. The diode D1 ensures that the transistor does not become reverse biased.
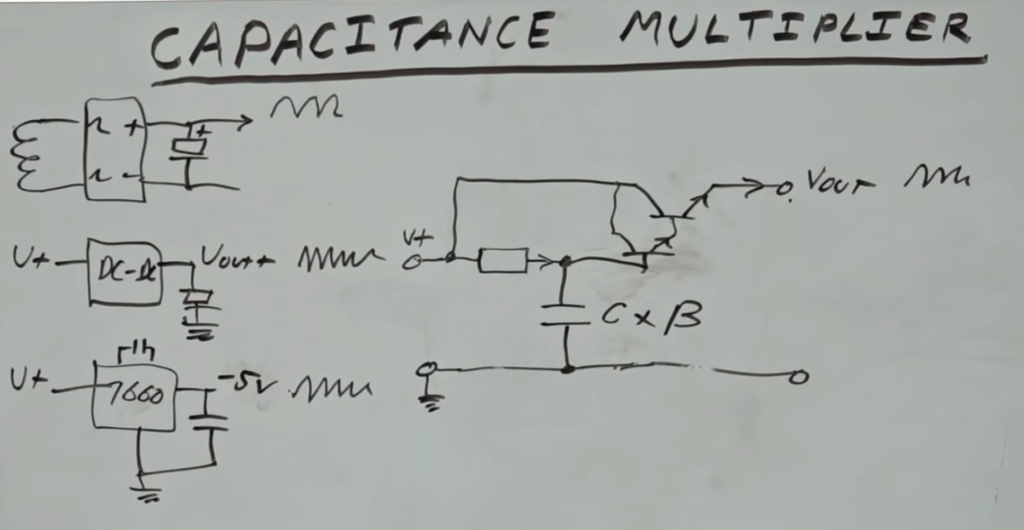